Highlights
-
Achieves high-capacity transmission of 336 Tb/s with a single light source
-
Eliminates the need for hundreds of built-in light sources in conventional transponder modules
-
Uses optical comb and frequency reference distribution technologies to generate 650 high-quality, frequency-synchronized carrier/local oscillator pairs over most of the S, C, and L transmission bands
-
Expected to accelerate commercialization and lower the costs of wide-band optical communication systems, including the S band
An international research team led by the Photonic Network Laboratory of the National Institute of Information and Communications Technology (NICT, President: TOKUDA Hideyuki, Ph.D.) demonstrated a coherent optical fiber communication system with a total transmission capacity of 336 Tb/s. The system uses a single light source combined with optical comb generation and frequency reference distribution, eliminating the need for hundreds of built-in light sources within transponder modules.
In this research, 650 sets of high-quality, frequency-synchronized pairs of carriers and local oscillators were generated over most of the S, C, and L transmission bands, through comb generation on the transmitter and receiver sides. Each comb line complies with the 25 GHz frequency standard of the International Telecommunication Union (ITU) and is qualified for dual-polarization 16-quadrature amplitude modulation (QAM) coherent optical communications. Optical frequency reference distribution was used to synchronize the two comb units.
This work will accelerate the commercialization of S-, C-, and L-band optical communication systems without the need for commercially available compact S-band light sources and will help to reduce the cost by simplifying the systems.
The results of this experiment were accepted as a post-deadline paper presentation at the 47th Optical Fiber Communication Conference (OFC 2024) presented by Ben Puttnam on Thursday, March 28, 2024.
Background
To cope with increasing data traffic demands, wavelength division multiplexing (WDM) and space division multiplexing have been investigated for high-data-rate optical fiber communications. NICT has demonstrated multiband WDM transmission with a total bandwidth of 37 THz by using all the major transmission bands of standard optical fibers. However, multiband WDM in a conventional optical communication system requires hundreds of compact, frequency-stabilized light sources within transponder modules. These light sources are currently not available for the S, O, E, and U bands.
Achievements
In this work, 650 sets of carrier/local oscillator pairs were generated over most of the S, C, and L bands (16 THz frequency band) through optical comb generation on the transmitter and receiver sides (see Figure 1). Each comb line complied with the 25 GHz frequency standard of the ITU and possessed sufficiently high quality (noise characteristics) for dual-polarization 16-QAM multimode fiber coherent communications. We also distributed an optical frequency reference to synchronize the two separate comb units on the transmitter and receiver sides. Consequently, each carrier and corresponding local oscillator automatically had the same oscillation frequency without needing independent frequency stabilization, as is the case for conventional coherent communication systems (see Figure 2).
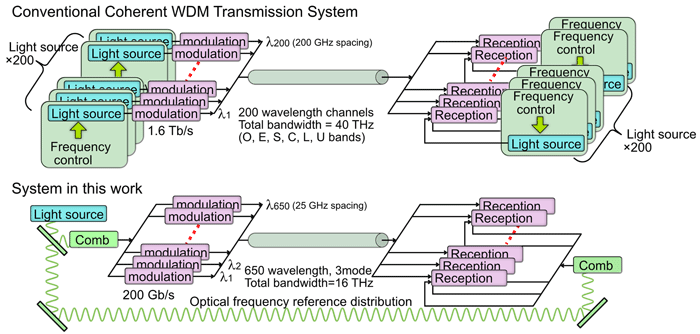
Figure2: Comparison of 320 Tb/s-class optical communication systems based on the conventional and proposed schemes. The conventional system requires 200 state-of-the-art commercial transponder modules with built-in light sources, ranging from the O to U band (total 40 THz), whereas the proposed system requires only one light source.
We used a 39-core multicore fiber with 38 cores supporting three-mode propagation and 1 core supporting single-mode propagation. One of the three-mode cores was used for data transmission and the single-mode core was used for distributing the optical frequency reference. The total transmission capacity was 336 Tb/s, which was almost 200 times greater than the data rate of the state-of-the-art commercial optical transponder module (1.6 Tb/s). If we were to deploy a commercial optical communication system with the same transmission capacity using conventional methods, we would need 200 transponder modules, including independent built-in light sources over the O, E, S, C, L, and U bands (40 THz frequency band). In this demonstration, however, we only needed a single light source instead.
The results of this experiment were accepted as a post-deadline paper presentation at the 47th Optical Fiber Communication Conference (OFC 2024, 24 to 28 March 2024) and were presented on Thursday, March 28, 2024.
Future prospects
This technology will eliminate the need for developing and implementing S-band built-in light sources and so will accelerate the commercialization of multiband WDM communication. The simple configuration (one light source) and automatic frequency locking between carriers and local oscillators will contribute to cost saving. Although we used only one of the three-mode cores in the 39-core fiber, full use of spatial channels (cores) will introduce further cost saving in optical communication systems.
References
International Conference: Optical Fiber Communications Conference (OFC) 2024, Post Deadline Session
Title: Wideband S, C,+ L-Band Comb Regeneration in Large-Scale Few-Mode MCF Link with Single-Mode Seed Channel
Authors: B. J. Puttnam, D. Orsuti, R. S. Luis, M. S. Neves, M. van den Hout, G. Di Sciullo, G. Rademacher, J. Sakaguchi, C. Antonelli, C. Okonkwo, L. Palmieri, and H. Furukawa
Previous NICT Press Releases
- “World Record 402 Tb/s Transmission in a Standard Commercially Available Optical Fiber”
https://www.nict.go.jp/en/press/2024/06/26-1.html - “World Record Optical Fiber Transmission Capacity Doubles to 22.9 Petabits per Second”
https://www.nict.go.jp/en/press/2023/11/30-1.html