Highlights
-
Record data-rate of 301 Tb/s in a standard commercially available optical fiber
-
27.8 THz optical bandwidth achieved by developing new optical amplifiers and optical gain equalizers for new wavelength (E) band
-
Significant contribution to the capacity expansion of optical communication infrastructure to meet the expected demand from new data-services
Researchers from the National Institute of Information and Communications Technology (NICT, President: TOKUDA Hideyuki, Ph.D.), in collaboration with Nokia Bell Labs, Aston University, and Amonics PLC, demonstrated a record-breaking data-rate of 301 terabits per second in a commercially available standard optical fiber.
This record was achieved by developing new optical amplifiers and optical gain equalizers to open up new wavelength bands that are not yet utilized in deployed systems. The newly developed technology is expected to make a significant contribution to expanding the communication capacity of the optical communication infrastructure as new data services rapidly increase demand.
The results of this experiment were accepted as a post-deadline paper at the European Conference on Optical Communication (ECOC) 2023 and presented by Ben Puttnam on Thursday October 5, 2023 at the Scottish Event Campus (SEC), Glasgow, UK.
Background
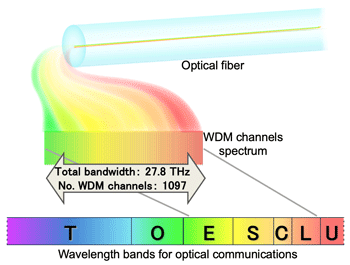
Figure 1 Wavelength bands used in optical communications
To meet the demand for enhanced data services, multi-band wavelength division multiplexing (WDM) technology using additional spectral windows for optical fiber transmission has been widely investigated. Adopting new transmission windows offers a potentially significant benefit in the near-term as a method of extending the life of already deployed optical fibers to provide additional transmission capacity without the large capital expenditure associated with new fiber deployment. However, moving away from the low-loss window of standard single-mode fibers (SMFs) requires new devices and amplification schemes beyond the standard erbium-doped fiber amplifier (DFA) that is a staple of C or C/L-band systems. Previously, S/C/L-band transmission has been explored with various amplifier technologies supplementing erbium DFAs including semiconductor optical amplifiers, Raman amplification and thulium-DFAs. NICT previously showed that combining DFAs with distributed Raman amplification has enabled 244.3 Tb/s transmission covering 19.8 THz over 54 km of SMF and more than 1 Pb/s when using a 20 THz bandwidth signal in a 4-core fiber with standard cladding diameter.
In this demonstration, we further expand dense wavelength division multiplexed (DWDM) transmission to include the E-band to enable more than 1,000 parallel (DWDM) transmission channels with 27.8 THz (212.3 nm) optical bandwidth.
Achievements
Along with collaborating partners, NICT constructed the world’s first E to L-band transmission system capable of DWDM transmission in a commercially available standard optical fiber supported by the development of new bismuth-doped fiber amplifier (BDFAs) and multi-port E-band optical processors (OP) for gain equalization (Details shown in Figure 3). The BDFA was used along-side thulium- and erbium- doped fiber amplifiers (T/E-DFA) together with distributed Raman amplification. In addition to the BDFA, the experiment required use of a prototype E-band gain equalizer to shape the E-band transmission spectrum.
A wideband DWDM signal comprising up to 1,097 channels covering 212.3 nm (27.8 THz) from 1,410.8 nm to 1,623.1 nm over the E, S, C and L-bands was transmitted up to 150 km. High data-rates were achieved by using dual polarization (DP-)QAM utilized with up to 256 symbols per constellation. As highlighted in Table 1, the data-rate after 50 km transmission with implemented LDPC decoding was 301 Tb/s, which exceeds the previous highest single-mode fiber (SMF) data-rate by over 23% and the near continuous transmission bandwidth of 27.8 THz is also a 41% increase. The generalized mutual information (GMI) estimated data-rate of 321 Tb/s is compared with past achievements in wideband transmission experiments in Figure 4. These results show the potential of E-band transmission, enabled by a new BDFA and multi-port OP, to increase the information carrying capability of new and deployed optical fibers.
Table 1 Table comparing previous wideband transmission demonstration
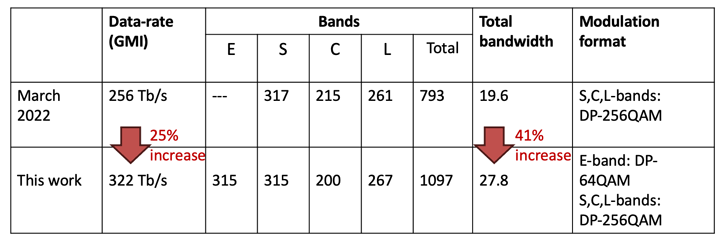
It is expected that the data-rate of optical transmission systems required to enable Beyond 5G information services will increase enormously. New wavelength regions enable deployed optical fiber networks to perform higher data-rate transmission and extend the useful life of existing network systems. It is also hoped that new bands can address the increasing demand of next generation communications services by combining with new types of optical fibers.
The paper containing these results was presented at the European Conference on Optical Communication (ECOC) 2023, one of the largest international conferences related to optical communications, having been selected as a post-deadline paper. The post-deadline session is a special session at the end of the conference to showcase the latest important research achievements and was held on Thursday October 5 at the Scottish event campus (SEC) in Glasgow, UK.
Future Prospects
NICT will continue to promote research and development into new amplifier technologies, components and fibers to support new transmission windows for both near and long-term applications. NICT will also aim to extend the transmission range of such wideband, ultra-high-capacity systems and their compatibility for field deployed fibers.
Reference
49th European Conference on Optical Communications (ECOC) 2023, Post Deadline Session
Title: 301 Tb/s E, S, C+L-Band Transmission over 212 nm bandwidth with E-band Bismuth-Doped Fiber Amplifier and Gain Equalizer
Authors: Benjamin. J. Puttnam, Ruben. S. Luis, Yetian Huang, Ian Phillips, Dicky Chung, Nicolas K. Fontaine, G. Rademacher, Mikael Mazur, Lauren Dallachiesa, Haoshuo Chen, Wladek Forysiak, Ray Man, Roland Ryf, David T. Neilson, and Hideaki Furukawa
Previous NICT press releases
- World's First Successful Transmission of 1 Petabit per Second in a Standard Cladding Diameter Multi-core Fiber
https://www.nict.go.jp/en/press/2022/05/30-1.html - Demonstration of World Record: 319 Tb/s Transmission over 3,001 km with 4-core optical fiber
https://www.nict.go.jp/en/press/2021/07/12-1.html