National Institute of Information and Communications Technology
Home > Press Release > World's First Demonstration of Space Quantum Communication Using a Microsatellite
- A big step toward building a truly-secure global communication network -
July 11, 2017
Highlights
- Successful demonstration of quantum communication between a satellite and a ground station
- A big step toward the development of future satellite constellations using small satellites
- It facilitates the adoption of this technology in a global truly-secure communication network
NICT developed the world's smallest and lightest quantum-communication transmitter (SOTA) onboard the microsatellite SOCRATES. We succeeded in the demonstration of the first quantum-communication experiment from space, receiving information from the satellite in a single-photon regime in an optical ground station in Koganei city. SOTA weighs 6 kg and its size is 17.8 cm length, 11.4 cm width and 26.8 cm height. It transmits a laser signal to the ground at a rate of 10 million bits per second from an altitude of 600 km at a speed of 7 km/s. We succeeded in correctly detecting the communication signal from SOTA moving at this fast speed. This is a major step toward building a global long-haul and truly-secure satellite communication network. As a result of this research, NICT demonstrated that satellite quantum communication can be implemented with small low-cost satellites, which makes it possible to many research institutions and companies to use this key technology. It is an achievement that opens a new page in the development future global communication networks, being a big boost to the space industry. The results of this research were accepted to be published in the prestigious peer-reviewed journal "Nature Photonics" (August 2017 physical issue, electronic version accessible from July 11, 2017).
※ This work was supported in part by the ImPACT (Impulsing PAradigm Change through disruptive Technologies) Program of the Cabinet Office of Japan.
Background
The technologies required to launch small satellites at a low cost have progressed immensely during this century, and significant efforts are being made to develop satellite constellations to achieve a global communication network covering the entire Earth. However, there is a need for a technology that can transmit large amounts of information from the space to the ground in short periods of time, and the current RF bands are already congested, setting a bottleneck of communication capacity. By using lasers, satellite optical communication has a readily-available frequency band and can transmit with higher power efficiency and with smaller and lighter terminals. Thus, it is expected to be a key technology to support the future satellite communication networks. Quantum communication, and more specifically, Quantum Key Distribution (QKD) is another key technology to guarantee the information security of the next global communication networks. Current QKD links are limited to several hundreds of km, thus implementing satellite-to-ground QKD is a fundamental step in this endeavor. QKD research is actively conducted in Japan, China, Europe, Canada and the United States (see supplementary information on recent research and development trends). In August of 2016, the University of Science and Technology of China launched a large (635 kg) quantum-communication satellite and performed a quantum-entanglement experiment with two ground stations.
Achieved results
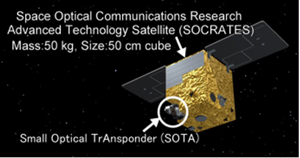
Fig. 1. Image of SOTA onboard SOCRATES.
SOTA is the world's smallest and lightest quantum-communication transmitter (6 kg weight, 17.8 cm length, 11.4 cm width, and 26.8 cm height) embarked on the microsatellite SOCRATES (see Fig. 1). SOTA transmitted two polarization states, encoding ‘0’s and ‘1’s (see Fig. 2a, b), to the ground at a rate of 10 million bits per second. The signals from SOTA were received at the NICT optical ground station in Tokyo's Koganei city, using a 1-m telescope (see Fig. 2c) in order to collect the transmitted photons and guide them to the quantum receiver (see Fig. 2d) to decode the information using a QKD protocol.
The signal that arrives at the 1-m telescope is extremely weak, with an average of 0.1 photons per received pulse. NICT developed the technology to perform the time synchronization and polarization reference frame matching between the satellite and the ground station directly from the QKD signals, as well as a quantum receiver capable of detecting such a weak signal with low noise. We demonstrated the world's first quantum communication from a 50-kg microsatellite. This will enable the development of future secure links from space by using quantum cryptography to completely prevent information leakage.
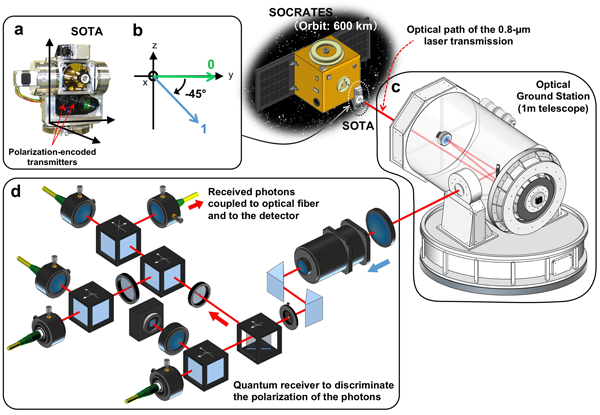
Fig. 2. Outline of the microsatellite SOCRATES and the NICT optical ground station located in Koganei city.
a. Picture of the lasercom terminal SOTA.
b. Polarization states that encode the bits of the transmitted information.
c. Optical ground station.
d. Configuration diagram of the quantum receiver.
b. Polarization states that encode the bits of the transmitted information.
c. Optical ground station.
d. Configuration diagram of the quantum receiver.
Future prospects
The technology developed in this project demonstrated that satellite quantum communication can be implemented by using low-cost lightweight microsatellites. Therefore, it is expected that many research institutes and companies, which are interested in this technology, will accelerate the practical application of quantum communication from space. In addition, since it was proved that long-distance communication is possible with very-low electric power, this will open up a path to speed up deep-space optical communication with exploration spacecraft.
In the future, we plan to further increase the transmission speed and improve the precision of the tracking technology, to maximize the secure key delivery from space to ground by using quantum cryptography enabling a truly-secure global communication network, whose confidentiality is currently threatened by the upcoming development of quantum computers.
・Journal: Nature Photonics (August issue, 2017)
・Title of the paper: Satellite-to-ground quantum-limited communication using a 50-kg-class micro-satellite
・Authors: Hideki Takenaka, Alberto Carrasco-Casado, Mikio Fujiwara, Mitsuo Kitamura, Masahide Sasaki, and Morio Toyoshima
・DOI: 10.1038/nphoton.2017.107
Video of the satellite quantum-communication experiment
Video of the satellite quantum-communication experiment
Relevant past press release
Supplementary Information
Trends on satellite quantum-communication technology
The technologies necessary to launch small satellites at a low cost have advanced immensely in the last few years, and by launching a big number of satellites into low Earth orbits, global communication networks covering the entire Earth in the form of satellite constellations are becoming a reality. These constellations will have to deal with a huge amount of data to be transferred to the Earth in short periods of time (since the typical pass of a LEO satellite is several minutes). Besides, the RF technology is becoming obsolete, and the radio spectrum congested. Satellite optical communication has a readily-available spectrum and the potential to increase the amount of transmitted data while reducing the power, mass and weight of the terminals.
Space lasercom has been demonstrated in many missions, mainly in Japan, Europa and the US. In May 2014, NICT developed a small lasercom terminal (SOTA) and launched it onboard the microsatellite SOCRATES into a 600-km sun-synchronous orbit. NICT successfully performed a variety of lasercom experiments, and since 2016 a new campaign of quantum-communication experiments was carried out.
Quantum communication is an essential technology for realizing quantum cryptography, which can completely protect the cryptographic-key exchange from any information leakage. Satellites can significantly increase the range of QKD links since the losses are smaller than when using optical fibers, which is typically limited to around 200 km, allowing intercontinental secret-key exchanges.
In August of 2016, the University of Science and Technology of China launched a large (635 kg) quantum-communication satellite and performed a quantum-entanglement experiment with two ground stations (J. Yin et al., Science, 356(6343), June 2017). The Chinese team is also working on experiments on intercontinental-scale quantum cryptography using this satellite (E. Gibney, Nature, 535, 2016).
Satellite laser communication and quantum communication are emerging technologies with a very big potential in the future global-scale communication networks, and they are attracting a great deal of attention from many important research institutions all over the World.
NICT Optical Ground Station and experiment results
Fig. 3 shows a diagram of the NICT Optical Ground Station(OGS) with a detail of the 1-meter telescope and the quantum receiver used in the experiments with its location in the telescope.
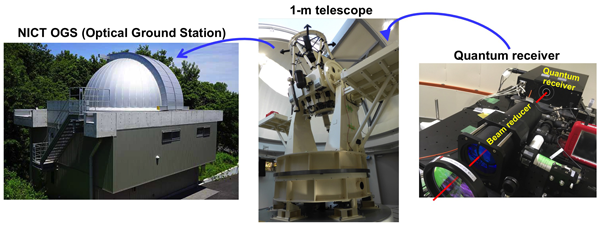
Fig. 3. Images of the NICT Optical Ground Station, the 1-meter telescope and the quantum receiver.
The signals transmitted from SOTA suffer from big losses and most of the transmitted photons are lost before reaching the receiver because of the divergence of the laser beam and the limited aperture of the telescope to gather the photons. Additionally, many photons are scattered and absorbed in the atmosphere. As a result, the signal arriving at the OGS is extremely weak, carrying an average of less than 0.1 photons per pulse. Since such weak signals cannot be detected by using conventional photodetectors, the quantum receiver used extremely-sensitive detectors, known as photon counters since they can detect single photons. This enables a much-higher efficient communication than conventional satellite optical communication. Also, by using signals with less than one photon per pulse, quantum cryptography allows to detect the presence of an eavesdropper, which makes it possible to deliver secret keys in a confidential way.
In order to realize quantum communication and quantum cryptography with such a weak signal, a key step is to accurately time-stamp the signals, so that they are clearly recognized in the quantum receiver. Therefore, it is necessary to accurately synchronize the signals between SOCRATES and the OGS to detect the transmitted bits without errors. It is also necessary to carry out a polarization-axis matching, because the reference frames change, due to the relative motion between the satellite and the ground station. Only Japan and China have been able to demonstrate these technologies in space, but China did it by using a 600-kg-class satellite, while Japan did it by using a 50-kg-class satellite.
Since the satellite moves at a fast speed relative to the OGS (about 7 km/s), the wavelength of the laser signal shifts due to the Doppler effect to a shorter wavelength when approaching the OGS, and to a longer wavelength when moving away from the OGS. Because of this Doppler effect, it is necessary to carry out an accurate time synchronization to be able to correctly detect the long sequences of bits without errors. In the China quantum-communication experiment, this synchronization was realized by using a dedicated laser transmitting a synchronization signal. By contrast, NICT was able to carry out this synchronization by using the quantum signal itself. A special synchronization sequence of about 32,000-bits was used in the quantum-communication signal for this purpose, and the quantum receiver was able to perform not only the quantum communication, but also the synchronization and the polarization-axis matching directly, by using only the weak quantum signal. In this experiment, NICT succeeded in demonstrating for the first time that quantum-communication technology can be implemented in small satellites.
Fig. 4 shows the SOCRATES orbit, as well as the Doppler-shift calculation and measurement of the experiment conducted on August 5, 2016. As shown in Fig. 4a, SOCRATES flew over the Pacific Ocean from the south to the north and reached the closest distance of 744 km to the NICT optical ground station at 22:59:41 Japan time. A communication link was established for 2 minutes and 15 seconds around that time. Fig. 4b shows the theoretical value of the Doppler shift predicted from the SOCRATES orbit information, and Fig. 4c shows the experimental value. The observed value of the Doppler shift showed a good agreement with the theory, and the change of frequency due to the Doppler shift could be accurately corrected. Based on this frequency correction, the time synchronization between the satellite and the ground station was established while accurately correcting the change of the time interval of photons coming from SOCRATES every second.
*Map: Edited version of a map by the Geospatial Information Authority of Japan, http://cyberjapandata.gsi.go.jp/#5/38.754083/137.109375/&ls=std%7Cmodis&blend=0&disp=01&lcd=modis&vs=c0j0l0u0t0z0r0f0. Map mosaic obtained from https://lpdaac.usgs.gov/data_access maintained by the NASA Land Processes Distributed Active Archive Center (LP DAAC), USGS/Earth Resources Observation and Science (EROS) Center, Sioux Falls, South Dakota, (Year). Source of image data product.
After establishing the time synchronization, the photon signal is transformed into ‘0’s and ‘1’s information bits. However, due to the bit-position shift, it is still necessary to match the bit sequence transmitted from SOTA with the bit sequence received at the OGS. As shown in Fig. 5, by analyzing the cross-correlation of the synchronization sequence of about 32,000-bits, this match could be successfully performed. Fig. 5b shows the correlation peak at the 29,656th bit position, which means that this is regarded as the origin in the OGS, so that the sequence can be correctly decoded.
Fig. 6 shows an example of a histogram of the series of detected photons by the quantum receiver. The Tx2 and Tx3 signals show the transmitted photons by SOTA, and the histogram shows how the detected photons are related to the original signal. This demonstrates that the synchronization could be accurately established by directly using the quantum signal, even in the presence of big losses.
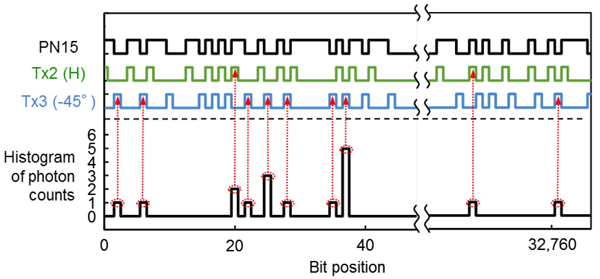
Fig. 6. Sequence pattern of the synchronization signal and the pulses transmitted from SOTA, and histogram of detected photons at the ground station.
Since SOCRATES is moving in relation to the ground station, the polarization reference frame between SOTA and the OGS is constantly changing. For a quantum-communication link to be established correctly, the polarization reference frame must be the same. If this relative change is not corrected, the polarization states corresponding to ‘0’s and ‘1’s cannot be accurately identified. Fig. 7 shows the predicted polarization angle of the photons transmitted from SOTA for ‘0’s and ‘1’s, as well as the measured angles, reaching a good agreement between both. The theoretical prediction was calculated by using the orbital information of SOCRATES, as well as its attitude change during the pass over Japan. By matching the reference frame, a quantum bit error rate as low as 3.7% could be measured. This demonstrates that quantum communication is feasible from space, since it is below 10%, frequently used as a condition for quantum cryptography to be secure, this being the first time such a demonstration has been performed by using a 50-kg-class microsatellite.
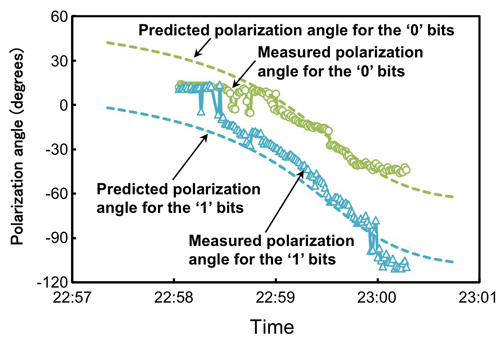
Fig. 7. Experimental result of the polarization-axis matching.
A good agreement is observed between the predicted and the measured polarization angles for ‘0’ and ‘1’ bits. The predicted values were calculated using the SOCRATES orbital and attitude information.
Glossary
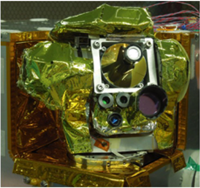
SOTA mounted on SOCRATES
SOTA(Small Optical TrAnsponder) is a compact optical communication device with a 5-cm telescope, a total mass of about 6 kg, a length of 17.8 cm, a width of 11.4 cm, and a height of 26.8 cm (see the image on the right). NICT conducted optical communication experiments from space to ground using lasers at several wavelengths.
AES, Inc. (President & CEO: Tadahiko Yoshida) developed a 50-kg class, 50-cm cube microsatellite for lasercom demonstrations with SOTA. SOCRATES stands for Space Optical Communications Research Advanced TEchnology Satellite. It has a three-axis attitude control and orbit position determination function by GPS receiver. It was launched on May 24, 2014 as a piggy-back satellite on an H-IIA rocket with the aim of establishing a standard microsatellite bus technology applicable to missions of various purposes. After the launch, it was safely injected into a 600-km sun-synchronous LEO orbit and finished its basic function bus test.
Quantum communication is a new technology based on the fundamental laws of quantum mechanics. It makes it possible to transmit information using a transmission power which is several orders of magnitude smaller than ordinary optical communication by encoding one bit per transmitted photon. In a broader sense, it also includes quantum cryptography, which reliably allows the transmission of cryptographic keys in a truly-secure way by being able to detect eavesdropping. Other applications, such as quantum teleportation, are also included in the category of quantum communications.
According to quantum mechanics, light combines the properties of waves and particles. Light particles are called photons and are the smallest units of light energy, which cannot be divided any further. For example, at a wavelength of 1.5 microns, which is usually used in optical communications, the energy of one photon is extremely small: ~10-19 Joule, or ~0.0000000000000000001 Joule. Single-photon regime means that the transmitted signal is emitting single photons, encoding one bit of information in each photon.
Quantum entanglement is a special state in which two or more fundamental particles, such as photons and electrons, are bound with a special correlation. What happens to one of the particles will determine instantly what happens to the other one, no matter how far they are from each other. This strange behavior has been demonstrated in many experiments. Quantum-entanglement distribution refers the transmission of a quantum-entanglement state between two distant places.
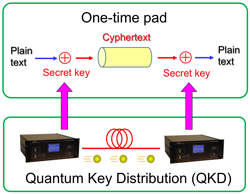
Quantum cryptography basic diagram
As shown in the figure on the right, quantum cryptography consists of sharing a secret key by using quantum key distribution (QKD) and one-time pad encryption. In QKD, the sender emits photons with the encoded bits of the cryptographic key, and the receiver detects the quantum state of each photon, eliminating the possibility of eavesdropping (in a process called key distillation). Thus, a secret key (which has to be a random number) is shared between both parties, the sender and the receiver. The Heisenberg's uncertainty principle is applied in order to be able to discover eavesdropping from a third party, in which case the shared cryptographic key is discarded.
Technical Contact
・Related to quantum communications
Masahide Sasaki
Quantum ICT Advanced Development Center
Advanced ICT Research Institute
Tel: +81-42-327-6524
E-mail:
・Related to satellite communications
Morio Toyoshima
Space Communications Laboratory
Wireless Networks Research Center
Tel: +81-42-327-5825
E-mail:
Media Contact
Sachiko Hirota
Press Office
Public Relations Department
Tel: +81-42-327-6923
Fax: +81-42-327-7587
E-mail: